Imagine your body as merely a vessel to an immortal crew. This crew is equipped with all the knowledge needed to navigate this survival machine through time and space, along with all the tools needed to survive, with a sole mission to ensure their safe passage to the next host. Ideally, the next body will be sturdier and better equipped to have a higher chance of surviving the next journey. If they fail, billions of years of evolution will all have been in vain. Welcome to the world of true time-travelers. Your body is a survival machine, crewed by genes in your DNA.
How Genes Code for Life
Genes govern all life on our planet. They provide instructions for the creation, maintenance and repair of your body, the survival machine. This machine can mean the human body, but also the body or cell of every animal or organism that ever lived. The genetic information stored in our DNA has traveled over billions of years in evolutionary history to reach us now, changing and adapting along the way, improving with each version.
Nucleotide Bases and DNA
Genetic information is stored in a very simple format. There are 4 basic building blocks that genes use, in the form of 4 nucleotide bases: adenine, thymine, guanine and cytosine. Adenine and guanine belong to a group of molecules known as purine, while cytosine and thymine are pyrimidines. Both purines and pyrimidines are widely found in nature. Caffeine, for example, is a purine, sharing a similar structure to guanine.

The 4 bases are better described as 2 pairs because adenine binds to thymine, and guanine to cytosine. Two strands of nucleotide bases often find themselves bound to each other by a multitude of pairings, becoming what we know as deoxyribonucleic acid (DNA). DNA is a large molecule and is most stable in a ‘double helix’ conformation often portrayed in diagrams and symbols.

From DNA to Proteins to Life
As mentioned, the base pairs in DNA hold the key to life. The arrangement of bases represents a language that our cells can understand. We have machinery that can unzip this DNA, read the instructions inside, then produce the proteins we need to survive.
Every three groups from adenine (A), thymine (T), guanine (G) and cytosine (C) spell out the code or ‘codon’ for a particular amino acid. For example, the amino acid alanine is coded for by the 3 base pairs GCA, and glutamine is coded by CAA. There are 20 amino acids in total. Some of these amino acids are produced by our bodies naturally, while we obtain others from our diet.

These amino acids can be linked to form proteins, each with different functions. Proteins might be cytochrome enzymes that help with metabolism, or they might produce pigmentation for the color of our eyes, or they may silently work in the background as part of our cell signaling machinery.
All the processes and functions of our body are overseen by proteins. In this manner, everything our body (the survival machine) needs is encoded in our genes. Genes are passed down from our parents, who received them from their parents, going all the way back to the origin of life on Earth billions of years ago.
How Genes Influence Their Carriers
The Time-Traveling Gene
Genes, these set of instructions within our DNA, can be seen as time-travelers. From the very first self-replicating molecule, genes have made a multitude of improvements through generations of species, culminating in life on Earth today. We represent the successful versions, of course, because genes that have made a mistake along the way don’t usually survive long. Gene lineages abruptly end if they are not transferred to the next generation of survival machines.
In order to be successful, genes must be able to exert visible, tangible benefits on the host. Imagine if a spaceship had a faster engine or a thicker hull, it would be better equipped to travel through space. This outward expression of genes is known as a trait—or the phenotype—in the body of the host.
If a gene (or a group of them) can produce beneficial traits in an individual, it is able to increase the chances of its survival and reproduction. Beneficial genes in a bird, for example, might include those that code for sharper claws (hunting), or brighter feathers (mating). In humans, such genes may manifest in a lower risk of certain cancers, or the lack of wisdom teeth.
When the carrier dies, the specific set of instructions is lost forever. That is why reproduction occurs; so that the carrier’s genes can remain ‘immortal’ through a fitter host. Therefore genes that provide carriers the best chance to stick around long enough to reproduce are the most successful ones, most likely to be passed down to the next generation.

Mutating Machines
On rare occasions, DNA is not properly replicated and mistakes arise in certain genes. Base pairs can be erroneously added, deleted or substituted. There are mechanisms in place to repair or remove such errors, but they can fail and allow the mutant genes to slip through undetected.
Sometimes, these mutations don’t cause any change to the overall phenotype of the organism; these are known as silent mutations. Other times, they alter the function of a key process in the organism. This usually results in an overall detrimental effect, with the embryo terminating development or the individual born with genetic defects.
On extremely rare occasions, however, mutations to a gene give rise to changes that bring tangible benefits to an individual. In birds, for example, there might be a mutation that leads to much stronger claws. Beneficial mutations improve the viability of the animal’s body, increasing the chance of successfully transferring the crew of genes over to the next survival machine.
Of course, this wouldn’t be considered evolution unless the specific mutated genes that improve survivability are passed on as well. This requires that the mutant genes be found in gametes, the reproductive cells. Gametes in humans are the sperm and egg cells, responsible for the actual transfer and combination of genetic material.
Humans have known for hundreds of years that traits in the parent organism are more likely to be transferred to their offspring. Genetic modification of animals and plants through selective breeding has brought us docile, domesticated wolves (dogs!) and crops with higher yields. There is great interest in gene therapy these days that look to cure genetic diseases by altering the mutations that are responsible.
Your Body, The Survival Machine
The Gene-Trait Relationship
As mentioned, genes code for proteins, which in turn affect the processes and features of an individual, also known as traits. In birds, genes code for traits like feather color or claw sharpness. In humans, genes would code for hair color or handedness.
We must understand, however, that there is no single gene that precipitates ‘sharp claws’ or ‘bright feathers’. Rather, traits tend to arise from a combination of factors. That is not to say that a single gene—all other things being equal—cannot produce sharper claws in an individual. But often, multiple genes and environmental factors decide the final outcome, making a ‘sharp claw’ gene difficult to pinpoint.
The relationships between genes are so complex that trying to understand the big picture through individual genes is almost impossible. Sort of like trying to explain how a complex machine works by individual component separately. However, progress in bioinformatics has led to breakthroughs like genome wide association studies (GWAS) that work by linking sets of genes to specific traits.
So, why, then, do genes work together in the first place? Or do they, for that matter? It turns out that it is not enough to be the ‘best’ gene and hope to be consistently passed on to the next generation. All genes are inherently ‘selfish’ in that they care only for their survival, but must work together to produce a viable carrier that functions well as a whole.
Genes Forced to Work Together
We can take apart the DNA of an individual and analyze each gene solely, based on the traits that it confers. We end up with a list of ‘good’ and ‘bad’ genes; the good ones improve the organism’s chances of survival, whereas bad genes decrease these odds. You might be thinking that if genes were ‘selfish’, each would simply evolve to become the ‘best’ version of itself and hope that its carrier survives long enough to reproduce!
The problem lies in the situation that there is only one body carrying an abundance of genes, both ‘good’ and ‘bad’. A ‘good’ gene in the company of surrounding ‘bad’ genes that decrease the organism’s odds of survival might be thought of as unlucky, but if such a situation arises consistently then it cannot be considered a good gene at all. Even if the good gene conferred the best version of that trait, it wouldn’t be passed on to the next generation.
The crew in your body are forced to stick together, no matter the consequences. Either all of them make it out alive, or none of them do—there is no in-between. They are therefore forced to work together with the goal to sustain their body long enough for sexual reproduction to take place.
If genes could exit individually by, for example, being sneezed out, this would be the preferred mode of genetic information transfer. Like viruses looking for hosts to replicate, the best genes would simply ‘infect’ other hosts, ensuring their survival.
Alas, genes only work together because they have to. While it is the nature of genes to have a form of ‘selfishness’ embedded within them, they know that they are stuck. Being the crew of a mortal machine, they must learn to survive together, or not at all.
The last section of this post is inspired by the author’s interpretation of “Survival Machines” in ‘The Selfish Gene‘ by evolutionary biologist Richard Dawkins.
About the Author
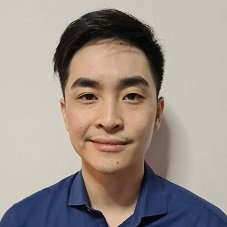
Sean is a consultant for clients in the pharmaceutical industry and is an associate lecturer at La Trobe University, where unfortunate undergrads are subject to his ramblings on chemistry and pharmacology.