Chemistry lessons—at both high school and college level—often begin with atomic structure and bond classification. This foundation proves inadequate for predicting reactions and breaks down entirely when used to explain more advanced physical chemistry concepts, such as quantum theory. By introducing electronegativity early on instead, we can build a solid foundation for chemistry education that showcases the importance of the electron; because chemistry is simply a game electrons play.
Problems in Chemistry Curricula Today
Understanding chemistry is beneficial, not just because you need to ace those exams, but because much of the natural world can be explained by chemical concepts. However, the current template for teaching chemistry restricts the student from grasping more complex theories; the problems lie in the manner atomic structure and bonding are introduced.
Why Atomic Structure is a Bad Starting Point
Many chemistry curricula use the structure of the atom as a starting point; protons and neutrons surrounded by orbiting electrons. Firstly, this is inadequate, as atomic theory is a hugely complex concept best described by quantum mechanics (even that doesn’t do a perfect job). However, teachers introduce a watered-down version of the theory as a stepping stone for future topics.
After papering over atomic theory, the concept of orbitals is often introduced, which describes how electrons in an atom are arranged around the nucleus (proton and neutron). Together, atomic structure and orbital theory lead students into a cul-de-sac of thought. You see, learning proton and mass numbers and numbering electrons in orbitals don’t tell us anything about molecular structure and reactivity—the cornerstones of (useful) chemistry!
For example, one of the first molecules we learn about is methane (CH4). Carbon has an atomic number of 6 (6 protons); hence it also has 6 electrons arranged in 1s, 2s and 2p orbitals. Since methane consists of four C-H bonds, the 2s and 2p orbitals of carbon are involved in bonding, as described in orbital theory.
Students are then thrown a curveball when taught that all four C-H bonds in methane are the same length, with the same energy, due to the hybridization of orbitals. They are often confused about hybridization because they have been taught that electrons exist in orbitals with 1, 4, 9 or 16 sub-orbitals, holding 2, 8, 18 or 32 electrons, respectively.
Since orbital theory cannot explain reality, we complicate it by saying the mixing of sub-orbitals occurs in hybridization. Worse, we don’t explain why and how this happens because it is governed by quantum mechanics (another concept that will surely confuse students later). Using atomic structure and orbital theory as a starting point for chemistry education simply doesn’t make sense!
Ionic and Covalent is a Poor Classification for Bonds
After students learn about atomic structure, they are often introduced to bonding being either ionic or covalent. They are taught that metals react with nonmetals to form ionic compounds (NaCl) while nonmetals react with nonmetals to form covalent compounds (CO2). Often, a comparison chart like the one below is drawn up:
Ionic Bonds | Covalent Bonds |
Between positive and negative ions | Between neutral atoms |
High melting points | Lower melting points |
Stronger bond | Weaker bond |
Dissolves in liquids to form ion pairs | Does not dissolve |
It is understandable to differentiate bonding between atoms as either ionic or covalent, as categorization is a natural human response to unfamiliar information. Students are much more comfortable if they can sort molecules into ionic and covalent, reactive or unreactive, soluble or insoluble. However, many of these properties in chemistry exist as a continuum rather than discrete parameters.
At best, this is a lazy way to introduce students to bond forming and chemical reactions since it makes them force-fit chemical bonds into one category or the other. Bonds exist as a spectrum, with fully ionic character on one end and weak dipole interactions on the other, with covalent interactions somewhere in between.
Fortunately, we can use a neat shortcut to address these problems in chemistry education and provide a strong foundation for learning future concepts. Bonding, hybridization, reactivity and other critical fundamentals of chemistry are built upon a single idea—chemistry is a game that electrons play.
Chemistry is a Game Electrons Play
Electrons and Electronegativity
Why focus on electrons? In chemical reactions, electrons are essential because they are all that the atoms and molecules ‘see’. Specifically, only the outer layer of electrons in an atom is involved in bonding, with the inner electrons and nucleus merely spectators (most of the time). If we can classify elements based on this, we would be able to make good predictions of molecular structure and reactivity!
It turns out that there is already a convenient tool available for us: The Periodic Table of the Elements.
Looking at the periodic table means we can immediately see the number of outer shell (i.e., reactive) electrons in each element (from 1 to 8 as we go across). Of course, the exception here would be the transition metals and heavier elements, as they don’t follow the same rules for the number of reactive electrons.
We can take this a step further to look at electronegativity values between these elements, which tend to increase from left to right and from bottom to top of the periodic table. Electronegativity is helpful because it measures the strength of an atom’s nucleus to draw electrons toward it. The electronegativity values and the number of reactive electrons in atoms gives us enough information to understand and predict a wide range of reactions.
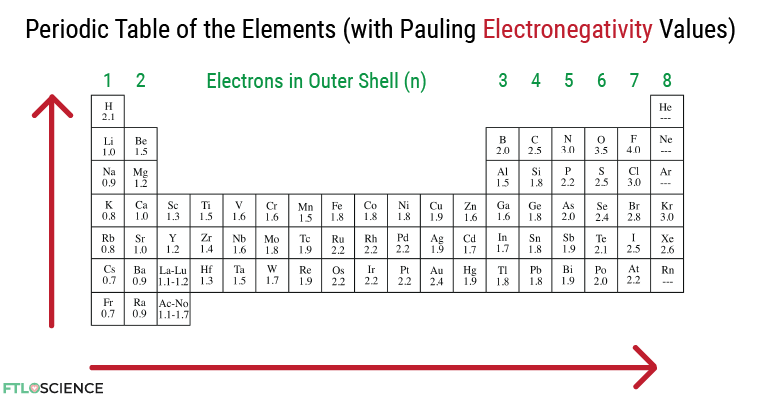
Let’s look at methane again (CH4). We know that the central carbon atom has 4 electrons in the outer shell because it is on the fourth column of the table. Next, we can look at the electronegativity of the C-H bond; 2.5 for C and 2.1 for H, with a difference of 0.4. This relatively small difference means that neither carbon nor hydrogen has an exceptionally high affinity to the electrons in their bond; hence the electrons are shared (i.e., covalent in character).
It is now clear to the student that the difference in electronegativity in atoms is what dictates if a bond is ionic or covalent, not the other way around. If there is a vast difference, the bond will likely exhibit ionic character. If the difference is slight, it will have covalent or dipole-dipole character.
However, electrons in molecules aren’t rigid and stuck between the bonds of atoms but are fluid across the entire molecule. Rather than looking at bonds in isolation, we can make better reactivity predictions when we think about electronegativities in the context of electron distribution.
Electron Distribution in Molecules
Electronegativity values, apart from being able to predict the type of bond that will form between atoms, can also predict the electron density of a molecule. In the C-H bond, carbon (2.5) has a higher electronegativity than hydrogen (2.1). This means carbon attracts electrons better than hydrogen, leading to a higher density of electrons around the center of the methane molecule.
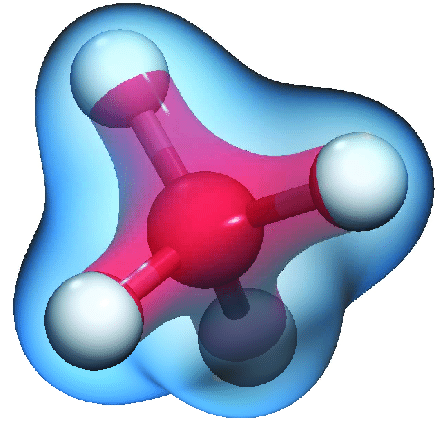
Let’s compare the difference in reactivity of a C-H and a C-F bond, with an electronegativity difference of 0.4 and 1.5, respectively. This means a larger dipole moment in C-F compared to C-H. Also, since F (4.0) has a higher value than C (2.5), we can also conclude that in this case, the electron density is centered around the F atom; the C in C-F is electron poor (i.e., an electrophile).
The electron density of a molecule also helps to explain reactivity. Since polarity is a vector, we must consider all bonds in a molecule before we can make statements on reactivity. Let’s compare CF4 and CH4, structurally similar, with the central C having four identical bonds to four identical atoms. In the case of C-F, the dipole is greater, as we mentioned above.
However, in both cases, the molecule’s symmetry means that all the dipoles cancel out—there is no dipole moment. Because of this, we can conclude that CF4 is more stable than CH4 because of the greater attraction of electrons to F in each of the symmetrical bonds (‘ionic’ vs. ‘covalent’). We’ve also explained their tetrahedral structure without utilizing orbital theory or hybridization!
Connecting Electronegativity with Quantum Chemistry
When quantum chemistry is later introduced to students, they learn that atomic orbitals are the result of electrons occupying the lowest energy states. These orbitals represent the space in which an electron is most likely to be found, calculated using the Schrödinger equation. When the energy difference between orbitals is small, they can mix to produce the lowest energy form (hybridization), a consequence of quantum mechanics (the linear combination of orbital wavefunctions).
However, this is at odds with what students have already learned, that only specific orbitals can mix (e.g., 2s+2p, 4s+3d, etc.). They often struggle to reconcile traditional atomic structure and orbital theory with the quantized energy states of atomic orbitals. This is another reason why teaching hybridization in isolation is detrimental.
Moreover, working knowledge of electronegativity helps the student grasp quantum effects in chemistry. The electronegativity values in atoms are calculated based on the scenario that their electrons are in the lowest energy state. Hence, when multiple atoms are involved, the resulting molecule will adopt the lowest-energy electron density configuration, with hybridization naturally arising.
Final Thoughts
Of course, atomic structure and orbital theory have their place in chemistry education (not quite sure about bond classification, though), for example in nuclear reactions and radioactive decay. However, they shouldn’t be used as the foundation for more complex concepts because they are irreconcilable with many of them. This disconnect can cause students to feel confused about chemistry, or worse, lose interest in the subject. Perhaps it is time to shift the focus in early chemistry curricula, because chemistry is simply a game that electrons play.
What do you think? If you’re a teacher or student of chemistry, it would be awesome if you shared your thoughts on this topic in the comment section below!
About the Author
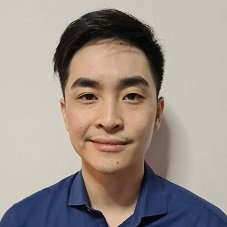
Sean is a consultant for clients in the pharmaceutical industry and is an associate lecturer at La Trobe University, where unfortunate undergrads are subject to his ramblings on chemistry and pharmacology.