Many of us would have heard about DNA and RNA, the two naturally occurring nucleic acid polymers that store genetic material. But we may not be quite as familiar with xeno nucleic acids (XNAs). The word is derived from the Greek ‘xenos’, meaning ‘stranger’ or ‘alien’. As one might infer, XNAs are a class of synthetic nucleic acids that aren’t found in nature. These structural analogs of DNA and RNA have many practical and theoretical uses, from helping scientists discover new drugs to providing insights into the origin of life.
A ‘Primer’ on DNA and RNA
Before we delve into xeno nucleic acids, it is helpful to understand the nucleic acids in our body, deoxyribonucleic acid (DNA) and ribonucleic acid (RNA). Nucleic acid polymers contain snippets of information known as genes, which are expressed in cells to synthesize proteins necessary for survival. They are also responsible for the safe storage and transmission of genetic material, which allows for cellular replication and sexual reproduction.
Nucleic acids are made of individual units, known as nucleotides, connected to form a long chain. Each nucleotide consists of 3 main components: A pentose (containing 5 carbons) sugar group, a phosphate group, and a nitrogenous base.

The phosphate and sugar groups are involved in connecting nucleotides with each other, forming a phosphodiester bond. Nucleotide polymers are also able to bind to each other, through hydrogen bonds between the nitrogenous bases. Adenine always binds to thymine (or uracil in RNA) and cytosine to guanine. This process is therefore commonly referred to as ‘base pairing’ and occurs because of the complementary structures of each nitrogenous base pair.
The Strange World of Xeno Nucleic Acids (XNAs)
This foray into synthetic nucleic acids—or XNAs—falls within the scope of synthetic biology, a multi-disciplinary pursuit that treads the interface of biology and engineering. With DNA and RNA, there is very little to choose from between the sugar groups and nitrogenous bases. As a result, the structural and chemical variety of nucleic acids is limited. To increase the number of possible modifications to genetic material, scientists started creating synthetic versions of these nucleic acids.
Designing new nucleic acids sounds rather futuristic; however, ideas pertaining to creating synthetic versions of DNA have been around since the 1960s. And from as early as the 1980s, scientists have been developing such polymers in laboratories. By improving upon the therapeutic potential of natural nucleic acids, XNAs are being researched as potential gene therapies. Some scientists are even trying to determine if DNA and RNA are the only viable sources of genetic material, or if we can incorporate XNAs into our bodies as a form of genetic engineering.

Nitrogenous Base Modifications
Scientists have added chemical groups such as fluorescent molecules to the nitrogenous bases in DNA, which can serve as bioimaging agents. They have also substituted entire natural groups (A, T, G, C, U) with new ones, creating synthetic nucleotide pairs that participate in ‘unnatural base-pairing’. These new base pairs can form new hydrogen bonds that occur in different patterns to those of natural base pairs, or form entirely new hydrophobic bonds instead1,2.
The introduction of several new, unnatural base pairs has certainly redrawn the boundaries of genetics, greatly increasing the potential information that can be encoded by nucleic acids. A drawback is that many of these unnatural base pairs are unable to interact with natural nucleic acids, limiting their usefulness. Future XNA developments will surely address this, forming exciting new interactions with natural nucleic acids present in living organisms.
Altering the Sugar and Phosphate Groups
The pentose sugar or phosphate groups can be modified to create XNAs too. Modifications in these two groups can change the structure of nucleic acids to improve their stability. Additionally, as the nitrogenous base remains unchanged, these XNAs usually retain their ability to interact with natural nucleic acids.
RNA differs from DNA only by a single hydroxide (-OH) group on the 2’ carbon atom of the pentose sugar. This change triggers subtle differences in properties, as seen in DNA’s increased stability compared to RNA. Scientists have experimented by substituting other species such as fluorine and oxygen-methyl (O-Me) groups at varying positions on the pentose sugar group3. Such substitutions are also employed in medicinal chemistry, altering the rate at which drugs are broken down by enzymes such as cytochrome P450.

Both major and minor changes to the phosphate group have also been studied. Replacing one of the oxygen atoms in the phosphate group with sulfur forms compounds known as phosphorothioate nucleotides4. Peptide nucleic acids (PNA) are formed by replacing the entire sugar-phosphate backbone with an N-(2-aminoethyl) glycine backbone, altering the properties of the entire molecule5. Complete replacement of the pentose sugar molecule, such as with glucose-like six-membered rings, has also been attempted.
Useful Applications of XNAs
The development of xeno nucleic acids is driven by certain drawbacks of natural nucleic acids. One key disadvantage of using DNA and RNA in biological applications is their vulnerability to nucleases, enzymes that are widely present within cells. Under normal circumstances, nucleases provide cellular immunity, where they recognize and remove foreign nucleic acids, such as those in viruses. However, nucleases can be quite a nuisance in some applications, as they degrade nucleic acid agents too quickly, preventing them from carrying out their desired effects.
Modifications are often introduced in XNAs to inhibit nuclease recognition and degradation, which allows XNAs to remain active and stable for a much longer time as compared to natural nucleic acids. With all this modification of genetic material, you might imagine scientists using XNAs to create strange creatures with other-worldly appearances and abilities. Fortunately, most scientists are a practical (and ethical) bunch who develop XNAs for use as therapeutics and in biotechnology research.
Aptamers
Aptamers are relatively short (15-100 nucleotide units) nucleic acid polymers that are designed to interact with a target, which can be another nucleic acid, a receptor protein, or even a small molecule. As biological drugs, they can serve as biomarkers, signaling the presence of molecules that are specific to a disease. Aptamers can also have therapeutic effects, triggering a response when they bind to their drug targets.
Therapeutic XNA aptamers work by suppressing or activating biological processes, such as inhibiting viral enzymes involved in virus replication. Modification of these aptamers offers the ability to improve their stability and target-binding efficacy. Macugen was the first aptamer drug approved by the American Food and Drug Association (FDA) in 2004, for the treatment of wet age-related macular degeneration. Macugen is actually a 27-unit XNA aptamer, manufactured by U.S. pharmaceutical company OSI Pharmaceuticals.
Small Interfering RNAs (siRNAs)
Small interfering RNAs (siRNAs) are tiny (~20-25 units) double-stranded RNA molecules that can bind to cellular RNA and alter gene expression, usually suppressing its activity. The small size and simplicity of its design make siRNAs ideal for therapeutics, such as preventing the expression of oncogenes in cancer treatment. XNA-modified siRNAs can be altered to be more stable than natural siRNAs, also improving on other factors like solubility and specificity6.
Evolutionary Insights
Some scientists are developing novel XNAs to better understand how DNA and RNA came into existence. It is unclear how current forms of our genetic material evolved from more primitive origins to become exclusive to all known domains of life today. The logical, step-by-step approach used in the laboratory to discover and refine new nucleotides provide insights into possible paths nature might have taken in the evolution of natural base pairs. Possible ‘predecessor’ nucleotide molecules have also been identified7.

XNAs are capable of storing and transmitting genetic information, with some variants also able to undergo ‘evolution’ within a laboratory environment8. Such findings imply that other polymeric molecules could also have served as genetic material, with the DNA and RNA in our cells the product of chance rather than design.
Conclusion
We understand that what’s natural may not necessarily be the best, driving scientists to push the boundaries of what nature avails us. By studying these modified natural molecules, materials, and even whole organisms, we can advance our knowledge to better benefit mankind. Nucleic acids might form the building blocks of biology, but they are not an exception. Xeno nucleic acids can be useful both in basic and applied sciences, from the development of new therapeutics to revealing the origins of life.
This article was written by Denyse Chang via our Write For Us program.
Reference
- Piccirilli, J. A., Benner, S. A., Krauch, T., Moroney, S. E., & Benner, S. A. (1990). Enzymatic incorporation of a new base pair into DNA and RNA extends the genetic alphabet. Nature, 343(6253), 33-37. doi:10.1038/343033a0
- Morales, J. C., & Kool, E. T. (1998). Efficient replication between non-hydrogen-bonded nucleoside shape analogs. Nature Structural Biology, 5(11), 950-954. doi:10.1038/2925
- Pinheiro, V. B., & Holliger, P. (2012). The XNA world: Progress towards replication and evolution of synthetic genetic polymers. Current Opinion in Chemical Biology, 16(3-4), 245-252. doi:10.1016/j.cbpa.2012.05.198
- Phosphorothioate Oligonucleotides. (n.d.). Retrieved from https://www.sigmaaldrich.com/technical-documents/articles/biology/phosphorothioates.html
- Pellestor, F., & Paulasova, P. (2004). The peptide nucleic acids (PNAs), powerful tools for molecular genetics and cytogenetics. European Journal of Human Genetics, 12(9), 694-700. doi:10.1038/sj.ejhg.5201226
- Morihiro, K., Kasahara, Y., & Obika, S. (2017). Biological applications of xeno nucleic acids. Molecular BioSystems, 13(2), 235-245. doi:10.1039/c6mb00538a
- Hirao, I., Kimoto, M., & Yamashige, R. (2012). Natural versus Artificial Creation of Base Pairs in DNA: Origin of Nucleobases from the Perspectives of Unnatural Base Pair Studies. Accounts of Chemical Research, 45(12), 2055-2065. doi:10.1021/ar200257x
- Pinheiro, V. B., Taylor, A. I., Cozens, C., Abramov, M., Renders, M., Zhang, S., . . . Holliger, P. (2012). Synthetic Genetic Polymers Capable of Heredity and Evolution. Science, 336(6079), 341-344. doi:10.1126/science.1217622
About the Author
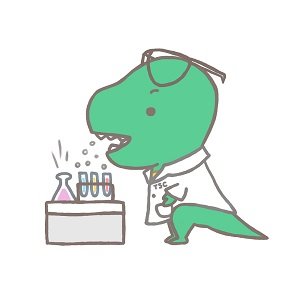
This article was written by a contributor. For a full list of guest writers, click here.