Nature is full of
While blue is the rarest color in nature, nobody really knows why. Some people think that it’s just pure chance that blue got left out of nature’s palette. Another theory is that the blue molecules are higher in energy than pigments of other colors, causing it to be unfavorable in the context of evolution.
The Color Blue in Nature
Blue is the Rarest Color
Of all the wavelengths of light that make up the electromagnetic spectrum, humans can only ‘see’ a small range of it. Yet, this so-called visible spectrum is enough to give us a remarkably vibrant perspective of reality! The machinery in our eyes and brain allows us to witness a continuum of color that makes the world around us much more enjoyable.
Even though blue is everyone’s favorite color, we scarcely see it in nature. Try it yourself! Close your eyes and think of the first blue animal that comes to mind. You see, most animals prefer a shade of brown or red for their coat or skin. Even when animals utilize coloration to stand out instead of camouflaging themselves, the color blue is rarely seen.
And out of the few animals with blue skin/feathers/scales, even fewer are ‘true’ blues. As you’ll see, many of these animals possess intricate surfaces that make them seem blue but actually contain no blue pigmentation at all!
No Pigment, Just Light Scattering
Arguably the most colorfully diverse group of animals, butterflies possess various colors in the most brilliant hues. This trait is especially apparent in blue morpho butterflies, with wings of iridescent blue. This bright blue is visible from long distances away, and can easily be picked up by a low-flying aircraft.
Indeed, you say, their marvelous wings must contain some blue pigment. Well, the answer is no. Scientists have found that—rather than pigmentation—tiny structures on their wings are responsible for their color. These microscopic scales reflect up to 75% of blue light reaching them, scattering them at large angles1. What about the other colors? What happens to them?

Remember that photons, being quantum objects, are better described as waves rather than individual particles. The scales on Morpho butterflies’ wings scatter blue light waves in phase, resulting in constructive interference. Other light colors are reflected out of phase, resulting in destructive interference between parallel waves. It ensures only the brilliant blue hue is observed at the physical level.
And this is just one of many examples of false blues in the natural kingdom. Birds that appear blue, such as bluebirds (duh), make use of tiny pockets of air in their feathers to scatter light. Furthermore, these birds often have feathers that absorb other non-blue wavelengths of light, causing a more pronounced and brilliant blue. Fish have the advantage of scales, which are suitable surfaces to produce iridescent colors. These reflective ‘tricks’ to create blue are comparatively more common in the natural world.
True Blues
In fact, the lack of blue pigmentation isn’t restricted to just animals and plants. Think about the blue of the sky, the sea, and even in blue eyes, all the result of physical phenomena! As an interesting aside, other eye colors (except for blue) do contain natural pigmentation. Just another example of the lack of blue pigmentation in our world.
But once in a blue moon, we find a living organism possessing this lesser-seen pigment. An example is the mandarin fish, with large swatches of blue skin. A quick look through a microscope reveals the presence of chromatophores (light-reflecting cells) on its skin containing actual blue pigmentation2! Another example can be found in the blood of horseshoe crabs, used in the pharmaceutical industry for detecting certain bacteria. Horseshoe crab blood contains hemocyanin, a protein that turns blue when bound to oxygen.

Why Natural Blue Is So Rare
Just Pure Chance
True blue or not, the color blue is nevertheless a rare natural occurrence. Colors play a huge role in nature; animals and plants use colors to attract mates, scare off predators and distinguish between individuals of a species. A rare color should confer an evolutionary advantage by making an individual stand out. Therefore, other factors must be in play to prevent the proliferation of blue.
One theory is that it is down to chance that blue isn’t in the ‘natural pool’ of colors. After all, most pigmentation in animals comes from their food. Flamingos are born grey and turn pink after consuming their signature diet of brine shrimp. Eating too many carrots and pumpkins (containing beta-carotene, a natural dye) will turn our skin a shade of orange! Hence the lack of blue pigmentation could be due to pure chance.
Another theory suggests that the lack of blue comes down to the costs of using this particular color. Not the cost in terms of money, but rather the energy cost. Energy is a valuable natural resource, where maximizing efficiency and effective conservation can be the difference between survival and death.
Blue Light has High Energy
From a chemical point of view, the emission of visible light results from fluorescence. Atoms and molecules contain electrons, which can be excited to higher energy levels; fluorescence occurs when these excited electrons return to their starting (ground state). Fluorescence is the excess energy in the form of a photon or light of a particular wavelength.
Each color-producing molecule (also known as a dye) requires different energies to excite its electrons. As it turns out, blue/violet light is of higher energy than other colors. This means that for a molecule to fluoresce a nice blue color, it must undergo a higher energy transition! For a compound to emit blue light, it must undergo a higher energy transition to do so. This means that the entire structure of the dye must also be of higher overall energy.

From an evolutionary perspective, having a bunch of high-energy structures that can emit blue light isn’t a particularly appealing prospect! These structures will require more energy to maintain and be more reactive than other lower energy, higher wavelength dyes, such as orange or brown. Even when animals and plants choose to present the color blue, evolution would gravitate toward using light-scattering physics instead of making these high energy pigments!
Reference
- Vukusic, P., Sambles, J. R., Lawrence, C. R., & Wootton, R. J. (1999). Quantified interference and diffraction in single Morpho butterfly scales. Proceedings of the Royal Society of London. Series B: Biological Sciences, 266(1427), 1403-1411.
- Goda, M., & Fujii, R. (1995). Blue Chromatophores in Two Species of Callionymid Fish. Zoological Science, 12(6), 811-814.
About the Author
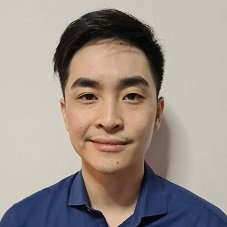
Sean is a consultant for clients in the pharmaceutical industry and is an associate lecturer at La Trobe University, where unfortunate undergrads are subject to his ramblings on chemistry and pharmacology.