If a single concept in chemistry underpins all others, it is electronegativity. This resource introduces electron behavior and density to beginners in a unique way, providing a fresh approach to building chemistry fundamentals. By the end of the guide, beginners will have mastered electronegativity and how to apply its principles to predict chemical reactions. After all, reactions are simply the movement of electrons between atoms—chemistry is just a game that electrons play.
Electronegativity builds a valuable foundation for chemistry! It’s not something we learn for the sake of learning (which, unfortunately, is the case for much of ‘education’). For beginners starting their chemistry education, we guarantee that mastering the concepts of electronegativity and electron density will significantly speed up the time it takes you to grasp new concepts.
Everything Revolves Around Electrons
Protons, Neutrons and Electrons
Atoms are the building blocks of chemistry, the Lego pieces that can be made into beautiful molecules. Atomic theory states that atoms can be further broken down into three components: protons, neutrons and electrons. The protons and neutrons are big, forming an atom’s core (or nucleus). The electrons, meanwhile, orbit around this nucleus.
Protons are positively charged, while electrons are negatively charged, so there must be an equal number of both. If an atom has 1 proton, it must have 1 electron and is the element hydrogen. If the atom has 2 protons, it has 2 electrons, forming helium. If 3 of each, lithium.
Neutrons are neutral (uncharged) and don’t always correspond to the number of protons and electrons. That’s all we need to know about atomic structure because—contrary to what many textbooks say—it’s not very useful for explaining chemical reactions.
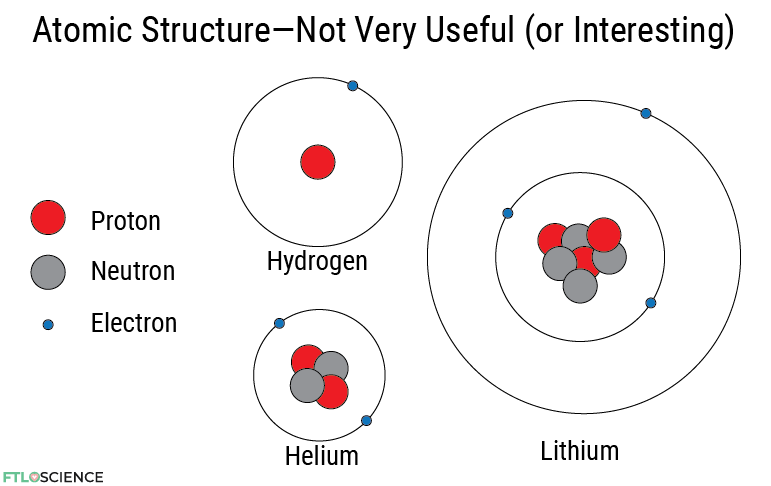
Atoms can come together to form molecules. For example, sodium (Na) and chlorine (Cl) react to form sodium chloride (NaCl), or table salt. Water consists of two hydrogen atoms and one oxygen atom (H2O). Bonding is essential in chemistry because all reactions either break old bonds, form new ones, or both.
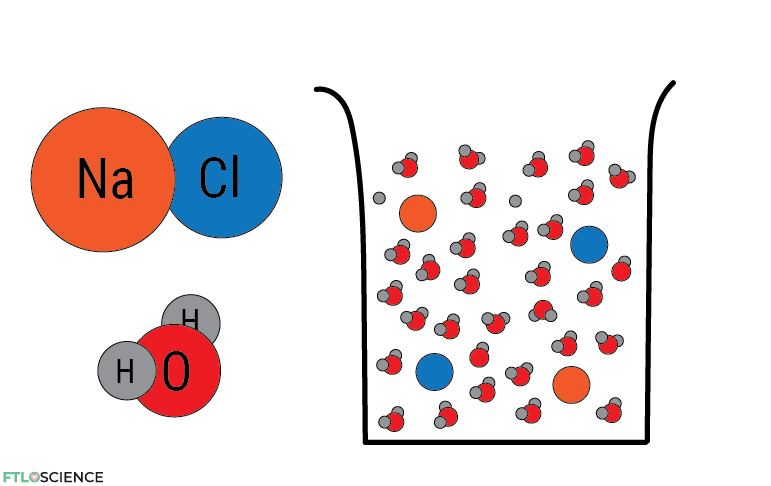
But how can I tell which atoms come together and which molecules move apart? There are so many possibilities! Unfortunately, atomic structure can’t help us out here. To answer this question, we must focus our attention on the tiniest particle in an atom—the electron.
Electrons and Electronegativity
Something that you rarely learn in class is that chemical reactions are simply the movement of electrons between atoms. The nucleus (protons and neutrons) isn’t involved at all! You can argue that we never really ‘touch’ anything since what we experience is the repulsion of our electrons with another object’s electrons.
Anyway, if we can predict how electrons move, we can become experts in chemistry! So how can we study this movement of electrons? (It’s actually more complicated than it sounds, but we can approximate!)
Let’s say you want to grab an electron off a hydrogen atom; the attraction between the positively charged nucleus and the negatively charged electron means a force is required. If there were no attractive force, then the electron would fall off like meat off a bone.
But because all atoms have at least one proton, they can attract an equal number of electrons. Factors that might alter this attractive force are the size of the atom and the overall number of protons. We call an atom’s ability to attract electrons its electronegativity (electron + negativity) value.
In atoms with high electronegativity values, the electrons are held more tightly to their core, while those with lower electronegativity values are happier to give up those electrons. Below is a periodic table with electronegativity values instead of mass numbers.
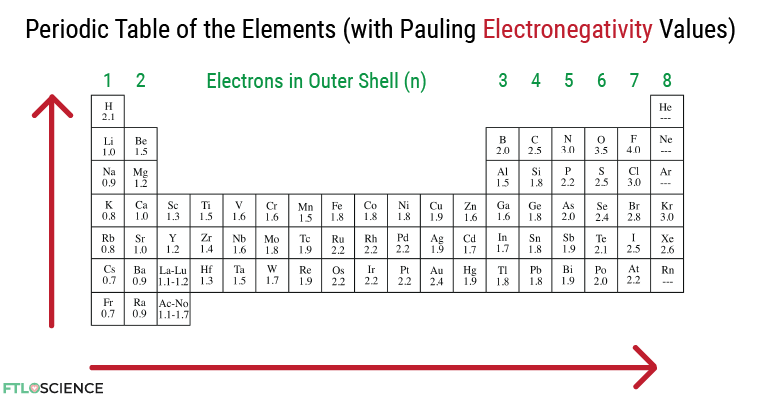
There is an overall trend that these values increase as we go from left to right of the periodic table—as the number of protons increases, a higher positive charge attracts the negatively charged electrons. Electronegativity also decreases as we go down the periodic table. As the overall size of the atom increases, the outermost electrons are further away from the core, hence experiencing a smaller force of attraction.
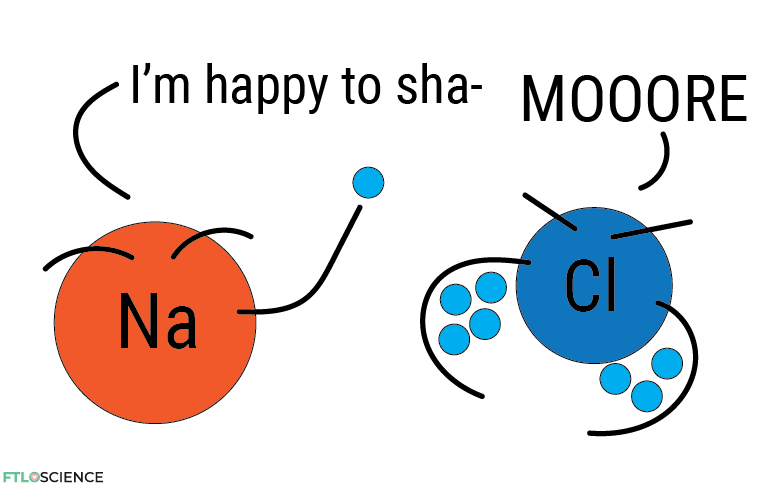
Mastering electronegativity begins with understanding the values of each element in the periodic table; that’s it! Now we can use these numbers to build a deeper understanding of molecules and reactions.
Using Electronegativity to Predict Bonding
Electronegativity values can also tell us about the strength of the bond. Suppose there is a significant difference in values between the atoms. In that case, the resulting bond will be strong since one atom will have a tighter hold on the other’s electron (i.e., ionic bonding). If the difference is slight, the bond will be weaker (i.e., covalent).
The periodic table of electronegativity is also helpful in predicting which elements have a stronger attraction or ‘pull’ when they come together as molecules. Carbon has a slightly higher electronegativity value in the C-H bond than hydrogen, so we expect the electrons to be closer to the carbon atom.
In the Na-Cl bond, the electrons are much closer to the chlorine than the sodium, so much so that they can be considered ions with opposing charges.
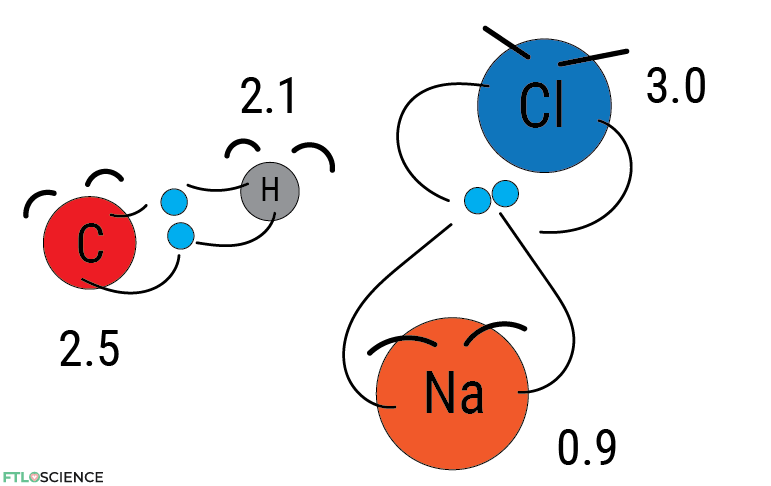
For those interested, the difference in electronegativities between any two atoms can be calculated using Pauling’s equation:
\left| \chi _{A}-\chi_{B} \right|=\\[0.1in](eV)^{-\frac{1}{2}}\sqrt[]{E_{d}(AB)-\frac{E_{d}(AA)+E_{d}(BB)}{2}}
The difference in electronegativities between atom A (χA) and atom B (χB) is calculated using the energy associated with the A-A, B-B and A-B bonds. A larger difference in χ signifies a stronger bond.
Electronegativity in Molecules
Energy Considerations
Another thing they don’t teach is that every reaction is the reduction of energy. This is also the case in chemical bonding, where electrons try to reduce their overall energy by looking for other atoms to bond with. The exception is the noble gases in the rightmost column of the periodic table have no electronegativity value—they are perfectly happy and stable.
Since the magic number is 8, other atoms can decrease their energy by getting their electron count to 8 (or 2 if it’s hydrogen). They achieve this through bonding, where electrons are shared between two or more atoms. We can use this to see how many bonds a particular atom can form; since carbon has 4 electrons, it needs 4 more to total 8, suggesting it can form 4 bonds. Nitrogen? 3. Fluorine? 1.
Hydrogen is unique in that only 2 electrons are needed to be considered stable (like helium), which is why hydrogen only forms a single bond instead of seven!
Electron Density and Molecular Dipoles
Electronegativities are also helpful for predicting which elements have a stronger attraction or ‘pull’ when they come together as molecules. In the C-H bond, for example, carbon (2.5) has a slight edge over hydrogen (2.1). We can expect the electrons to be closer to the carbon than the hydrogen, making it more likely to find an electron in the space around the carbon; it has a higher electron density.
We must remember that electrons are always on the move, but the probability of their location matters in the context of reactions. The regions where they are more likely to be found are considered to have high electron density, with a buildup of negative charge.
Conversely, areas with a low likelihood of being found are electron deficient, with a positive charge. We label them as delta positive (δ+) and delta negative (δ-) charges, indicating that they aren’t ‘formal’ charges—the molecule remains neutral despite the imbalance of electron density.
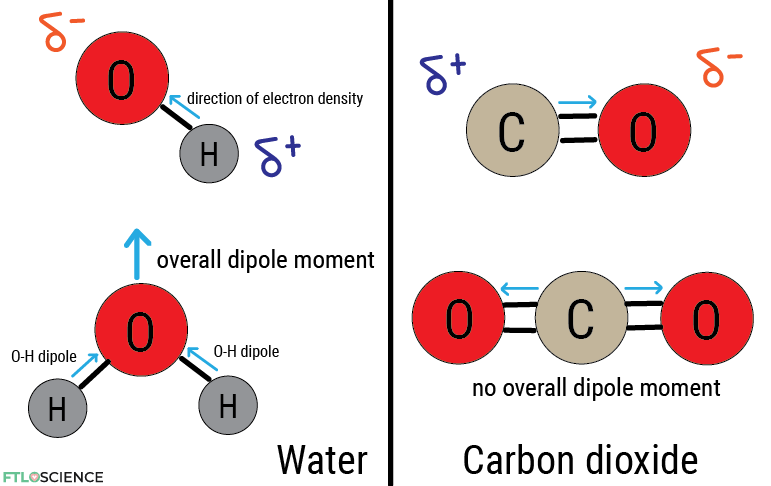
These electron density imbalances are called dipoles. When a molecule displays electron asymmetry, that is, when the electron density is pulled in a specific direction, it possesses a dipole moment. In water, where the electron density is pulled toward oxygen, the molecule’s shape means it has a molecular dipole that points toward the oxygen atom.
In carbon dioxide, its shape means that both oxygen atoms pull in opposite directions, canceling out the overall molecular dipole.
Using Electronegativity to Predict Properties of Molecules
Remember that chemistry is a game that electrons play, and their behavior is always dictated by energy. As an extension, molecular reactions are also at the whim of electrons and their energy concerns. Electrons in molecules are always looking for ways to balance their densities.
Regions of high electron densities will seek out areas of low electron density. In organic chemistry, these electron-rich regions are called nucleophiles, while the electron-poor regions are electrophiles.
The relative strengths of nucleophiles/electrophiles can be estimated by the difference in electronegativities of the atoms involved, combined with the resulting dipole. Let’s begin by comparing the reactivities of chloromethane (CH3Cl) with tetrachloromethane (CCl4).
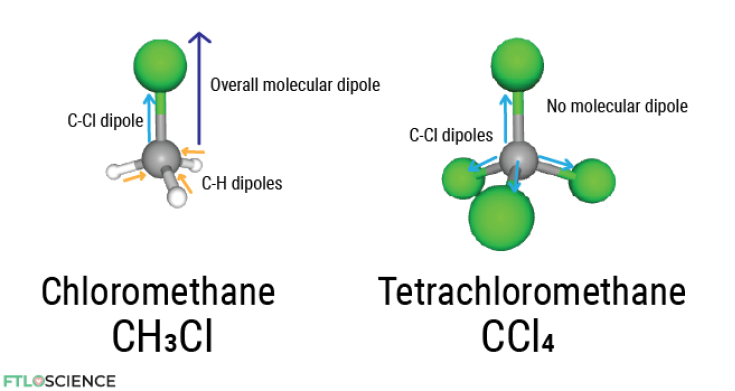
The C-Cl bond results in the electron density concentrated around chloride due to its higher electronegativity value (3.0 vs. 2.5 in carbon). In chloromethane, there is a strong overall dipole in the direction of the C-Cl bond.
Chloromethane is highly volatile, having a flash point of -20 °C. In the atmosphere, the gas has an atmospheric lifetime—the average time it persists in the environment when released into the atmosphere—of about ten months.
The same C-Cl bond is present in tetrachloromethane, but there is no overall dipole in the molecule since the chlorine atoms cancel each other out. It is so unreactive that the molecule was once used in fire extinguishers. However, tetrachloromethane is rarely used today as it is ozone-depleting and a greenhouse gas, with an atmospheric lifetime of a whopping 85 years!
Case Study Reaction: Nucleophilic Substitution to Produce Methylamine
Let’s look at chloromethane again. The high electronegativity value of chlorine means that the carbon center is electron poor (an electrophile). Electron-rich molecules (nucleophiles) are attracted to it, such as ammonia (NH3), with high electron density around the nitrogen atom. Remember that reactions are driven by imbalances in electron density! We can use arrows (in red below) to show the movement of these electrons.
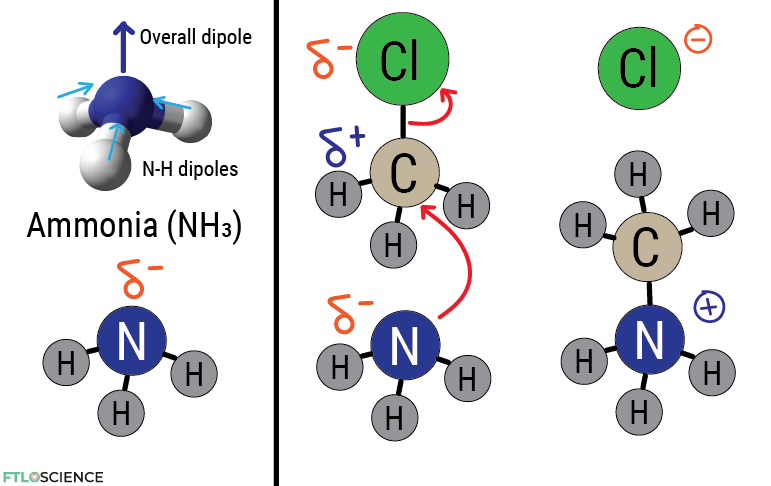
After ammonia binds to the carbon center, the intermediate molecule is imbalanced since carbon cannot have five bonds. As a result, the chlorine atom acts as the ‘leaving group’, grabbing the extra electron on carbon for itself and becoming a chlorine ion, no longer a part of the initial molecule. In organic chemistry, this is known as a nucleophilic substitution reaction.
We can denote the formal negative charge on the chlorine ion with a negative sign. The nitrogen is ammonia is now bonded to 4 other atoms, making it electron deficient (it doesn’t like sharing more than 3) and holds a positive charge. However, if more ammonia is left in the mixture, it can react with the positively charged ion, grabbing a proton so the electron can be returned to nitrogen.
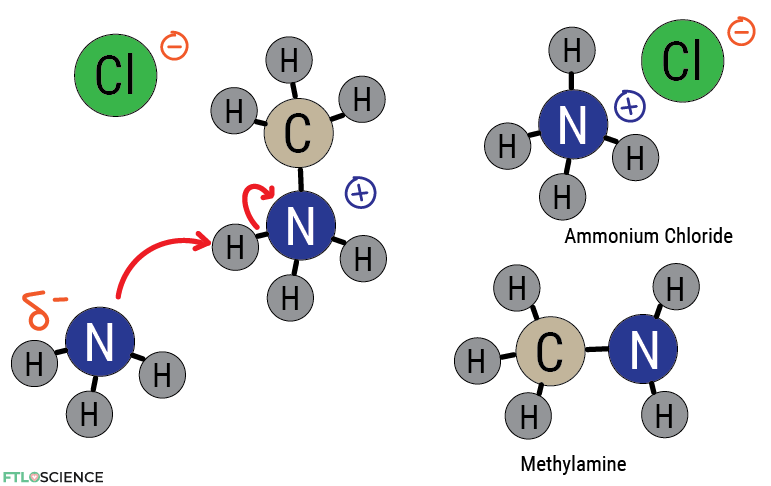
The result is the production of methylamine—a versatile reagent in organic synthesis (and a methamphetamine precursor). Electronegativity provides a valuable tool for predicting how molecules will react!
Further Considerations
Symmetry and Hybridization
One thing that is not taught in class is that electronegativity provides a surprisingly smooth transition into orbital theory and hybridization. In many introductory textbooks, hybridization is traditionally discussed in the context of mixing of ‘orbitals’ (s and p, s and d, etc.) to explain the identical lengths of C-H bonds in methane.
However, it is more intuitive to look at the electronegativity values. Since methane consists only of C-H bonds, this must mean that they share the same electronegativity difference and must all have identical bond strengths and lengths.
This principle applies to all other molecules that have hybridization of orbitals, decreasing the overall energy of all the electrons involved. Since electronegativity theory has this energy consideration built-in, there is no further need to alter it to explain hybridization.
Limitations of Electronegativity in Chemistry
Although it is a helpful tool for predicting bonding and reactions, electronegativity does have some limitations. Electronegativity was designed as a quick way to determine bonding and the nature of a bond without resorting to quantum mechanics and complicated calculations.
However, this doesn’t always reflect what occurs, as electrons are considered quantum particles and subject to quantum effects. As a result, some cases of bonding cannot be explained by electronegativity alone, especially in molecules with atypical bond angles.
Another glaring gap is its inaccuracy in predicting the bonding between large complex molecules where dipole moments are difficult to calculate. Since electrons must be thought of as shared across the entire structure of a molecule, it isn’t feasible to focus on the behavior in each bond separately.
There are also different ways to calculate electronegativity. The method discussed so far assumes Linus Pauling’s calculations (the original) which fit many models. Since then, chemists have found different ways to predict the values for the periodic table of electronegativity, using other parameters and data.
However, mastering electronegativity theory provides a robust foundation for further learning, making it a good starting point for chemistry beginners!
Reference
Chloromethane and tetrachloromethane 3D structures are sourced from PubChem
About the Author
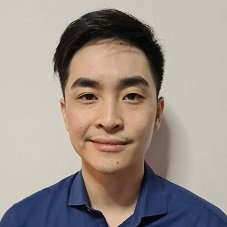
Sean is a consultant for clients in the pharmaceutical industry and is an associate lecturer at La Trobe University, where unfortunate undergrads are subject to his ramblings on chemistry and pharmacology.