The global monoclonal antibody market has an annual value of USD 100 billion, boasting blockbuster drugs such as Humira and Keytruda. Monoclonal antibodies are immune cells that have the ability to bind to very specific targets. By doing so, they can elicit a response against virtually any disease. Today, monoclonal antibodies are among the most successful candidates for the development of new drugs. How do they work? And what makes them so successful?
Basics of Monoclonal Antibodies
Our immune system consists of immune cells, working day and night to keep our body healthy. It works by removing pathogens – stuff that the immune system thinks will make us ill. These include viruses and bacteria, among other foreign species.
B cells play a big role in this, creating ‘tags’ that bind to pathogens, putting the rest of the immune system on alert to remove them. These ‘tags’ are known as antibodies. In order for antibodies to recognize a pathogen, it has to contain a very specific binding region. It is this binding region that allows it to bind tightly to a specific part of the pathogen known as an antigen.
An antigen can be a tiny protein or even a whole virus. At any rate, antibodies detect antigens. When our immune system is up and running normally, we do an excellent job producing a variety of unique antibodies. Vaccines, such as the ones against the coronavirus, take advantage of this technique to ‘boost’ our immune system with certain antibodies, without exposing us to the antigen itself.
But what if we could design our own ‘tags’ to specifically target diseases in the body? This idea that arose in the early 1980s inspired an entire field of research: therapeutic monoclonal antibodies (mAbs). The advantage of this strategy is that we can take advantage of the immune system to produce the required antibodies, bypassing the need for structural studies of the target antigen.
In principle, this means that we design specific mAbs to target and destroy any pathogen in the body, without damaging the rest of our cells. Sounds great, doesn’t it? Unfortunately, since the approval of the first monoclonal antibody therapy in 1985, they haven’t quite hit ‘magic bullet’ heights.
Evolution of the Monoclonal Antibody
Now, the B cells that we clone to produce monoclonal antibodies usually come from mice; human cell lines are comparably less stable. Now, a life-threatening problem arises when we try to introduce these mAbs into our bodies. Our immune cells can sense this foreign material and proceed to attack it—the immune system can be quite silly sometimes.
To combat this, researchers quickly began to replace bits of the mouse antibody with human fragments, to discourage the immune cells from destroying it. It was a challenge of chopping mAbs up and put them back together again, but this time, more human-like. However, these chimeric antibody therapies didn’t really take off, mainly due to adverse reactions and other side effects.

The real breakthroughs came about after humanized antibodies started to enter the field, in the early 1990s. These are essentially human antibodies, with the only mouse component being the antigen-binding regions. Other techniques (such as transgenic mammals and phage display) allow for the creation of fully human mAbs, virtually invisible to our immune system.

Other Shortcomings of mAbs
Another problem they face is poor bioavailability. As monoclonal antibodies are ‘large’ molecules compared to the usual small molecule drugs, they are not easily absorbed into our bloodstream. If we simply ingest mAbs orally, our liver can quickly metabolize them before they even get the chance to work.
This means that their administration has to be direct—straight into the bloodstream—instead of ingestion in the form of a pill. This usually takes the form of regular infusions, which can be expensive to the patient, not to mention painful.
But other issues also plague the development of mAbs. Since they are produced in huge vats of cells as opposed to chemical reactors, it is difficult to optimize their conditions for growth. This form of biologic drug also tends to have a wide range of impurities in the final product.
Apart from the host cell’s proteins and DNA, growth factors and hormones must be removed from the final mixture. Some of these, like bacterial endotoxins, present risks of serious adverse reactions in patients. Technologies that aid the development of mAbs are, therefore, a huge opportunity for future research.
Harnessing Their Potential
Since the first FDA-approved monoclonal antibody therapy in 1986, there are now 131 mAbs available on the market, with hundreds more in drug development pipelines. We know that monoclonal antibodies offer us the ability to target and bind to specific antigens, providing therapeutic benefits. But how can we develop successful drugs based on this technology?
There are several ways mAbs can be put to medical use. One of which is the testing for the presence of certain pathogens or substances, such as the SARS-CoV-2 virus, using enzyme-linked immunosorbent assays (ELISA). HIV detection and pregnancy test kits also fall under this umbrella.
In addition to diagnostics, mAbs binding can also alter inflammation and even cancer pathways. Since all processes in our body are governed by chemical binding, there are few limitations to the therapeutic potential of mAbs. We’ll explore some below.
Humira (adalimumab)
The anti-inflammatory mAb Humira (adalimumab) was brought to the market in 2002, for the treatment of rheumatoid arthritis. It works by binding and inhibiting tumor necrosis factor-alpha (TNFα), a key protein in the immune response. Patients suffering from rheumatoid arthritis usually have high TNFα levels, causing the immune system to attack their joints2. This painful autoimmune disease has no known cure, affecting tens of millions of adults worldwide.
Since then, Humira has been approved to further treat a wide range of autoimmune diseases. Despite its patent expiry, Humira maintains its superstar status, with Abbvie reporting sales of over USD 18 billion in 2017.
Keytruda (pembrolizumab)
Perhaps unsurprisingly, oncology is another key target for mAbs. Cancer cells tend to have certain proteins on their surfaces that bind to and inhibit the action of immune cells. If we can block this interaction, then the immune system can perform the dirty work of destroying the tumor. This is the mechanism behind Merck and Co.’s Keytruda (pembrolizumab).
Our immune cells possess programmed cell death protein 1 (PD-1) receptors, that serve to block immune action when bound. This is known as an immune checkpoint – a system that prevents the immune system from accidentally targeting them3. However, certain cancer cells take advantage of this by overproducing proteins that bind to PD-1, stopping immune cells from killing them.

Keytruda binds to and blocks the PD-1 receptor, preventing cancer cells from binding4. This allows the immune system to recognize the tumor as foreign, and initiate a response against it. Although Keytruda’s initial approval was for the treatment of melanomas, it is now indicated for other cancers that have certain genetic anomalies.
Future Drug Discovery
The specific and high-affinity binding of mAbs, compared to traditional small molecule therapies, make them attractive targets for drug discovery. Combine this with ever-advancing technologies for their development and production, and there is no doubt that monoclonal antibody therapies will continue to prosper.
In an ideal world, we will be able to screen targets for their unique epitopes, and efficiently design monoclonal antibodies that bind with high affinity to them. Through the regulation of receptors and signal pathways, virtually any disease has a direct cure. Perhaps Paul
Cover graphic: Rendition of parent B cells producing monoclonal antibodies by Melanie (@nanoclustering)
Reference
- Reichert, J. M. (2001). Monoclonal antibodies in the clinic. Nature Biotechnology, 19(9), 819.
- Jongen-Lavrencic, M., Peeters, H. R., Wognum, A., Vreugdenhil, G., Breedveld, F. C., & Swaak, A. J. (1997). Elevated levels of inflammatory cytokines in bone marrow of patients with rheumatoid arthritis and anemia of chronic disease. The Journal of
rheumatology , 24(8), 1504-1509. - Francisco, L. M., Sage, P. T., & Sharpe, A. H. (2010). The PD-1 pathway in tolerance and autoimmunity. Immunological Reviews, 236(1), 219-242.
- Le, D. T., Durham, J. N., Smith, K. N., Wang, H., Bartlett, B. R., Aulakh, L. K., … & Wong, F. (2017). Mismatch repair deficiency predicts response of solid tumors to PD-1 blockade. Science, 357(6349), 409-413.
About the Author
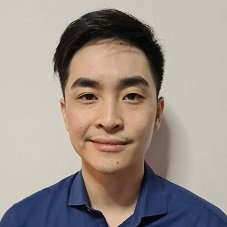
Sean is a consultant for clients in the pharmaceutical industry and is an associate lecturer at La Trobe University, where unfortunate undergrads are subject to his ramblings on chemistry and pharmacology.