While developing a drug product, we often overlook the chemical stability of the ingredients. Many drug components are susceptible to reactions like hydrolysis and oxidation, reducing the drug’s overall effectiveness before reaching the patient. How do we determine the shelf life of a drug, and how can we reduce chemical degradation?
The shelf life of a pharmaceutical drug product is calculated as the time it takes for 10% of the active ingredient to degrade. We can extend this by modifying the chemical structure to prevent reactions like hydrolysis and oxidation. We can also reduce the rate of decomposition with desiccants and proper storage.
Shelf Life of a Pharmaceutical Product
The shelf life of a drug is generally the time it takes for a 10% reduction in the active ingredient concentration (or 90% of it to remain). Sometimes, a more stringent 5% reduction is used as the shelf life (95% remaining).
The ideal shelf life of a drug product is between 1 to 5 years. Any expiry date within 1 year of manufacture poses logistics problems, while having a shelf life of more than 5 years doesn’t make financial sense. Many drug products still stable 5 years after manufacture are given an arbitrary expiry date within this range.
There are several ways drugs degrade, which we will discuss in the sections below. Stability tests are a vital component of the drug development process, as pharmaceutical companies must submit stability study data of all drug candidates before it can be approved for market.
Hydrolysis—Reaction with Water
Which Drugs Degrade by Hydrolysis?
Water is a reactive molecule, as the oxygen atom tends to be electron rich. Water is also everywhere in our environment, which makes it bad news for drug compounds. Chemicals undergo hydrolysis reactions, where water (hydro) molecules break their bonds (lysis).
Aspirin (acetylsalicylic acid) contains an ester group that is a prime target for hydrolysis. As the oxygen atoms attract electrons, the carbon atom between them becomes electron poor. As the reaction below shows, the electron-rich oxygen atom in water attacks the electron-poor carbon, forming salicylic acid and acetic acid.
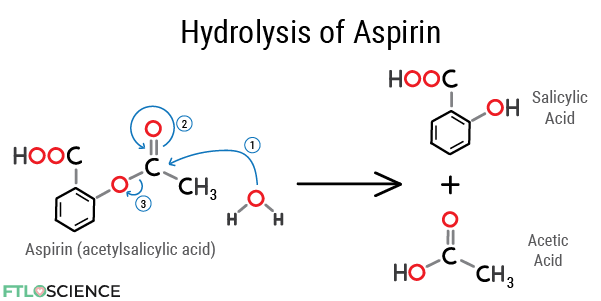
Other functional groups susceptible to hydrolysis include lactones (cyclic esters), amides and imides, all of which contain a leaving group with a high electronegativity value.
Drugs with a significant bond strain are more likely to undergo hydrolysis, such as penicillin. The beta-lactam (4-membered cyclic amide) ring makes the molecule a likely target for hydrolysis. When dissolved in water, penicillin undergoes 38% decomposition after 24 hours at room temperature, forming penilloic acid.
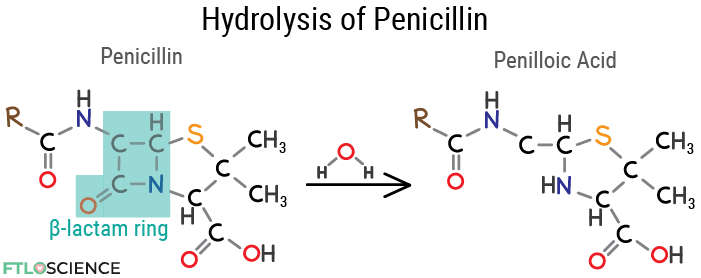
Penicillin breaks down faster under acidic conditions (like in the stomach), which is why many of this class of drugs must be intravenously administered. We can modify the chemical structure of penicillin drugs to be less susceptible to hydrolysis; amoxicillin, for example, is a penicillin-class antibiotic that can be taken orally.
Preventing Hydrolysis in Drug Products
Changing the chemical structure of a drug compound can prevent it from being hydrolyzed. The main driver for reactivity with water is the presence of electron-poor regions on the molecule. We can reduce this electronegativity difference by substitution with other atoms.
For example, amide bonds are structurally similar to ester bonds but have a lower electronegativity difference, which slows down the hydrolysis of the entire molecule.
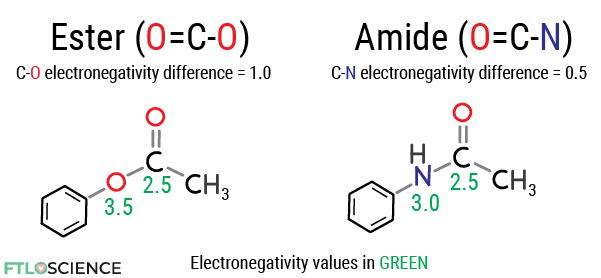
Proper pharmaceutical packaging can reduce the rate of hydrolysis in many drug products, especially single-use packaging like blister packs. Multi-use packaging exposes the contents to water vapor in the environment every time they are opened, which is why they are unsuitable for water-sensitive drugs. We often see desiccants like silica gel that can sequester water in these containers.
The temperature and humidity of the environment also play a role in the rate of hydrolysis, which is why we often see storage instructions on medicine bottles to keep them in cool and dry places.
Oxidation—Reaction with Oxygen
Oxygen Radical Reactions
The second most common form of chemical degradation occurs through oxidation reactions. As you might have guessed, these reactions occur because of the reactivity of oxygen in the air. Oxygen forms radicals that can kickstart various reactions, forming more radicals from drug products.
Hydroxyl (-OH) groups in certain alcohols, like glycerol (from fats), break down in the presence of oxygen to form carboxylic acids. Nitrogen and sulfur-containing groups also oxidize readily, as seen in the table below (source).
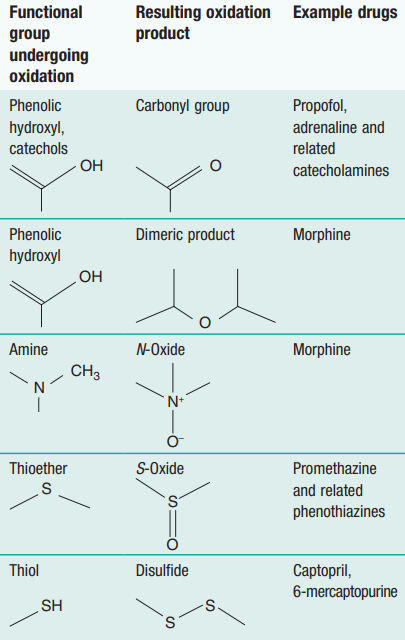
We can tell when a molecule has been oxidized by counting its number of bonds to oxygen atoms. For example, the single C-O bond becomes a C=O double bond when alcohols are oxidized into aldehydes. And when aldehydes oxidize to form carboxylic acids, oxygen bonds increase again (O-C=O).
Preventing Oxidation in Drug Products
If a drug is prone to oxidation, we can add antioxidants to its formulation ingredient list. Antioxidant (or reductant) molecules are much more reactive to oxygen than the drug ingredients, hence undergoing oxidation in place of them.
Antioxidants commonly added to drug formulations as preservatives include ascorbic acid (Vitamin C), sulfur-containing compounds, butylated hydroxyanisole (BHA) and butylated hydroxytoluene (BHT).
Preventing oxidation reactions can ultimately be challenging because of how much oxygen is in the atmosphere. Many types of plastic used as pharmaceutical packaging material are permeable to gases, which means oxygen can make its way into the drug product even if the container is sealed.
For drugs susceptible to oxidation, we need to use higher grades of coated plastics or glass containers that provide better oxygen barriers. We can also purge containers with an inert gas like helium or nitrogen to drive out ambient air that contains oxygen before sealing.
Heat-sealing technologies form an airtight seal around the mouth of containers that contain tablets or capsules, protecting the drug product from oxidation during storage and transport while forming a tamper-proof seal.
Other Degradation Pathways
Photodegradation—Damage from Light
Photons of light can also change the molecular structure of certain drugs. Depending on its wavelength, the photons reaching the surface of Earth from the sun take the form of either infrared, visible or ultraviolet light. Of these, ultraviolet (UV) light has the highest energy and causes the most damage to drug products.
Light can activate oxygen molecules, increasing the rate of oxidation. Direct photodegradation is also possible, where the energy from the light breaks bonds (photolysis) between molecules. Photolysis is most common in drug compounds containing carbonyl, nitro, alkene and aromatic functional groups.
The most common way to protect drug ingredients from light damage is to use opaque containers. Certain plastic materials are translucent or opaque, but glass can be colored by using additives that provide its contents with better protection from light. Metal containers are rarely used because of their high reactivity.
Reactions With Bottles/Containers
On the topic of containers, we need to ensure that the packaging in contact with the drug product isn’t reactive toward it. For example, specific plastic polymers are reactive toward organic molecules and can affect the stability and viability of the medicine.
Absorption into and adsorption to plastic container walls is possible, which can decrease the effective concentration of active ingredients and reduce the shelf life of the drug product. Choosing a suitable container is a key part of drug development.
Glass containers are usually inert, but leeching (molecules in the glass diffusing into its contents) can occur in lower-grade glass types. Metal containers are the most reactive, as metal atoms are generally electron poor. Metals can also act as catalysts for oxidation reactions.
Chemical Degradation of Biologic Therapies
Tertiary Structure of Proteins
So far, we’ve discussed chemical degradation for small molecules, but biologics—drugs created through biological processes—have various unique stability issues. Biologics like proteins are large molecules, meaning that certain parts of the structure interact.
These interactions contribute to the physical stability of the overall protein molecule, called its tertiary structure. This structure is crucial because it dictates the protein’s therapeutic effect. However, the bonds that hold the tertiary structure in place are not as strong as covalent bonding in small molecules.
Protein tertiary structures are often held together by hydrogen bonding, disulfide bonding, hydrophobic interactions and other electrostatic interactions that are considered ‘weak’ forces. These forces can be broken through:
- Heating and environmental fluctuations (hot/cold cycles)
- Interactions with other proteins (aggregation)
- Container absorption/adsorption
- Hydrolysis, oxidation and photodegradation
Once the structure’s shape is altered, the protein might not refold to form the original structure, hence losing its therapeutic effectiveness.
Controlling Protein Stability
One way we can reduce the chances of protein degradation is to replace the amino acid residues. Some amino acids, like cysteine (-SH), are reactive, so we can replace them with serine (-OH) to increase stability.
That being said, biologics generally require more specialized handling than traditional small molecule drugs. They are poorly soluble molecules that also readily undergo hydrolysis, which means they cannot be taken orally.
Protein therapies are usually administered as an IV infusion after reconstitution with water or saline. It is inconvenient to the patient and more expensive since they can only be stored in sterile type 1 glass containers.
Because of their chemical and physical instability, they also require controlled storage conditions, which complicate their storage and transport. Proteins are usually chilled or frozen during transport, which adds to the costs of producing such drug products.
Protein therapies also tend to have short shelf lives, sometimes just a few days after reconstitution. As more and more biologics are approved as therapeutics, we will need to develop improved methods to control their chemical stability and improve their shelf life.
About the Author
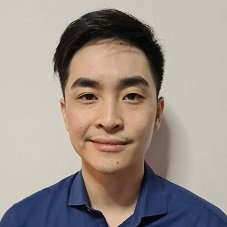
Sean is a consultant for clients in the pharmaceutical industry and is an associate lecturer at La Trobe University, where unfortunate undergrads are subject to his ramblings on chemistry and pharmacology.